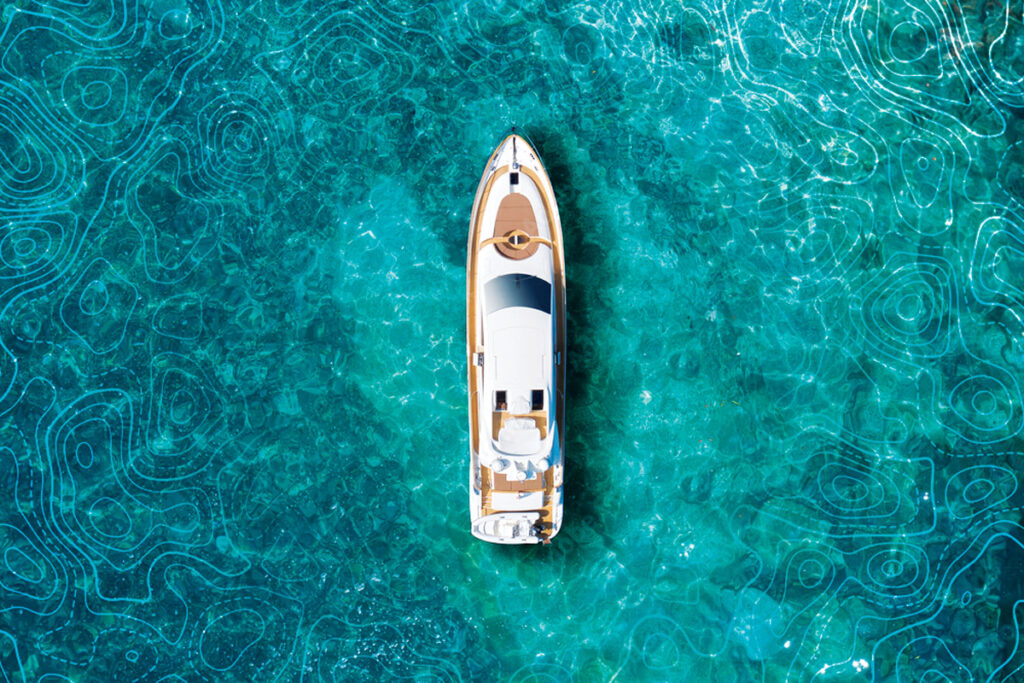
If you love nautical yarns, David Grann’s The Wager is a must-read. The nonfiction work details the account of The Wager, a sixth-rate Royal Navy square-rigger that carried 28 guns and 120 sailors. The 123-footer was part of an eight-vessel armada that, in 1741, sailed west around Cape Horn in winter, pursuing plunder. The Wager got separated. In a navigational blunder, the vessel turned north before it banked enough west. It didn’t go well.
Anyone interested in learning about how the great east-west navigation problem was finally solved should read Dava Sobel’s Longitude. Anyone interested in ensuring their own navigational accuracy, however, should cruise with a dedicated Global Navigation Satellite System sensor.
Satellite-based navigation began evolving in the 1960s. The US-built Global Positioning System—the first of its kind—went live in 1993. Today, the international Global Navigation Satellite System consists of four global satellite constellations (including GPS), plus two regional ones. While GPS continues to provide world-class service, GNSS receivers can capture this information along with data from other satellites. The best part? You probably already own several.
Navigational satellites work by broadcasting information about their identification, position, orbit and health status, along with a hyper-accurate time stamp. Receivers derive their position by triangulating with at least three satellites, with stronger (or more numerous) signals often equating to higher accuracy. Precision matters. According to the Federal Aviation Administration, if a clock aboard a GPS satellite is off by one-thousandth of a second, then the corresponding measurement error would be 1,616 nautical miles.
While there are differences between the various constellations, each system employs three distinct segments: control, user and space.
The control segment consists of one or more master land-based control stations and a global network of supporting stations. These stations monitor each satellite’s reported positions and compare reports with predictive models. If needed, operators can alter a satellite’s orbit to ensure baseline accuracy or avoid debris.
The user segment refers to any receivers listening for signals, while the space segment refers to orbiting assets.
Each of the GNSS’ four main navigation constellations contain different numbers of satellites that operate at different elevations and across different orbital planes. The US-built GPS constellation involves 31 satellites that operate at an elevation of 10,900 nautical miles above sea level. GPS satellites orbit on six different planes, and they maintain a 55-degree orbital inclination (relative to the equator).
Russia’s GLONASS constellation, which has been active since 1995, involves 24 satellites that operate at 10,315 nautical miles and orbit on three planes at 64.8 degrees of orbital inclination. China’s BeiDou constellation, which went live in 2011, consists of 35 satellites. Of these, eight are either geosynchronous or inclined geosynchronous satellites that operate at 19,325 nautical miles, while the other 27 operate at 11,625 nautical miles. BeiDou satellites orbit the planet on six planes, and they maintain an orbital inclination of 55.5 degrees.
Finally, the European Union’s Galileo constellation, which became operational in 2018, will (when complete) consist of 30 satellites that operate at 12,540 nautical miles. Galileo satellites orbit across three planes, and they maintain a 56-degree orbital inclination.
GNSS receivers are generally accurate from 6.5 feet to 13 feet globally; however, some countries use a satellite-based augmentation system (SBAS) that improves accuracy by broadcasting correction information. In the United States, this is called the wide-area augmentation system (WAAS). In early 2023, the European Union’s Galileo constellation began delivering free high-accuracy service information that’s precise to roughly 8 inches.
Celestial navigation, this is not.
Satellite-navigation receivers have existed in different forms for years. Depending on the manufacturer and design, these receivers (and their antennas) can be embedded into multifunction displays, or incorporated into abovedecks sensors that share satellite-navigation data with other networked equipment (or both).
Alternatively, yacht owners can sometimes buy an abovedecks listen-only antenna, which shares its improved signal strength and reception with a belowdecks GNSS-enabled multifunction display.
Some satellite-navigation receivers favor GPS signals, while others can also listen to data from some of the other GNSS constellations. Full GNSS receivers can access the four main constellations, plus the regional Japanese and Indian constellations. These receivers sometimes include nine-axis compasses or attitude-heading reference systems. These sensors, which don’t add much cost, provide accurate heading information in addition to GPS and GNSS data.
Finally, satellite compasses are the best option for serious navigation. Depending on their design, these instruments employ multiple GNSS receivers, which provide heading information that isn’t contingent on Earth’s magnetic field (read: high-latitude cruising). Eric Kunz, Furuno’s senior product manager, says the company’s SCX20/21 satellite compasses use four GNSS receivers, allowing them to achieve 1-degree heading accuracy.
While some people say GPS alone is plenty robust for their needs, many marine-electronics manufacturers have been quick to embrace GNSS. “With more satellites available to track and pull into calculations, the GNSS-enabled receivers offer enhanced accuracy,” says Jim McGowan, Raymarine’s Americas marketing manager.
He’s not alone in this assessment. “A GNSS antenna provides more redundancy and higher accuracy than GPS-only receivers,” says Dave Dunn, Garmin’s senior director of marine and RV sales. “Some parts of the world may have better coverage at certain times of day with some constellations than others.”
McGowan says GNSS is especially useful for high-latitude navigators because these receivers can track GLONASS satellites: “Those satellites are in a higher orbit inclination than GPS satellites, which allows the GNSS receiver to get a better tracking angle and duration on those satellites.”
Leigh Armstrong, Simrad’s product manager of digital systems, agrees: “This allows for better maintenance of accuracy in areas with less satellite coverage.”
The inverse, of course, is that BeiDou, Galileo and GPS satellites likely provide better fixes closer to the equator.
While GNSS data is critical for navigation, it can also help bolster the accuracy of other networked devices. Here, Dunn points to automatic identification system (AIS) position and speed data, autopilot performance, and radar target-tracking features.
Looking ahead, autonomous docking systems and vessels need precise position, speed and other navigational information to negotiate harbors, follow autopilot-driven courses, and safely dock. It’s expected that GNSS (with SBAS) will fill this niche.
The Wager’s crew experienced unspeakable horrors, but GNSS receivers and satellite compasses likely mean none of today’s boaters will have to dodge scurvy.
Belts and Suspenders
While the ancient mariner would have paid handsomely for a chronometer, contemporary smartwatches carry GNSS sensors. Most smartphones have GNSS receivers, as do some handheld VHF radios. These are all important backups should a vessel experience low voltage or power loss.